Fuelling
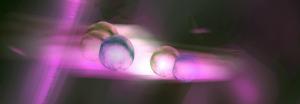
Hydrogen atoms, with a single proton, have the lowest electrical charge of all atomic nuclei and thus the smallest barrier to overcome. It turns out the cocktail recipe for fusion improves even more when you use hydrogen's isotopic variants deuterium and tritium. While other fuels could, in theory, be fused, they would require even higher temperatures than ITER's 150,000,000 °C.
Although different isotopes of light elements can be paired to achieve fusion, the deuterium-tritium (D-T) reaction has been identified as the most efficient for fusion devices. Only a few grams of fuel are present in the plasma at any given moment. While a 1000 MW coal-fired power plant requires 2.7 million tonnes of coal per year, a fusion plant with the same ouput will only require 250 kgs of fuel per year, half of it deuterium, half of it tritium.
Deuterium can be distilled from all forms of water. It is a widely available, harmless, and virtually inexhaustible resource. In every cubic metre of seawater, for example, there are 33 grams of deuterium. Deuterium is routinely produced for scientific and industrial applications.
Tritium is a fast-decaying radioelement of hydrogen that occurs only in trace quantities in nature. It can be produced during the fusion reaction through contact with lithium, however: tritium is produced, or "bred," when neutrons escaping the plasma interact with lithium contained in the blanket wall of the tokamak.
Lithium from proven, easily extractable land-based resources would provide a stock sufficient to operate fusion power plants for more than 1,000 years. What's more, lithium can be extracted from ocean water, where reserves are practically unlimited (enough to fulfill the world's energy needs for ~6 million years).
Global inventory for tritium is presently around twenty kilos, which ITER will draw upon during its operational phase. The concept of "breeding" tritium within the fusion reaction is an important one for the future needs of a large-scale fusion power plant.
Fuel cycle
The fuels used in ITER will be processed in a closed cycle. Less than 1g of fusion fuel is present in the vacuum vessel at any one moment.
As a first step to starting the fusion reaction, all air and any impurities must be evacuated from the vacuum vessel. The powerful magnets that will help to confine and control the plasma are then turned on and the low-density gaseous fuel is introduced into the vacuum vessel by a gas injection system. Once the fuel has been introduced into the vacuum chamber, an electrical current is applied to the system which causes the gas to break down electrically, become ionized, and form a plasma.
The gas injection system will provide the initial "fill" of the vacuum chamber prior to plasma initiation, it will puff gas into the chamber during the ramp-up phase, and it will enable the control of plasma density during the flattop of the plasma burn. The system is designed for a throughput of 200 Pa m³/sec on average and 400 Pa m³/sec at peak, almost one order higher than that of any existing tokamak.
A second fuelling system, a pellet injector, will also be used on ITER. An extruder punches out several millimeter-sized deuterium-tritium ice pellets that are propelled by a gas gun up to 3,600 km/h—fast and cold enough to penetrate deep into the plasma core. The frozen pellets are injected through a guide tube located in the inner wall of the vacuum vessel and another guide tube for outer wall injection. Pellet injection is used to control plasma density and is also efficient in controlling Edge Localized Modes, or ELMs (energetic bursts that escape the magnetic field surrounding the plasma and cause energy loss). Special technology is being developed to allow these pellets to fly along curved trajectories, thereby attaining specific zones within the plasmas where ELMs are particularly disruptive.
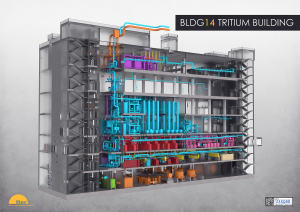
Tritium Plant
Fuelling the fusion reactions in ITER is not a "once-through" process; instead, fuel that is not consumed gets pumped out as part of torus plasma exhaust, together with helium ash and impurity gases, and recycled through the Tritium Plant for reuse. Although the effective burn rate in the plasma chamber is estimated at only 1%, that's enough for helium ash to begin accumulating and the core plasma to dilute. To avoid this, powerful torus cryopumps in the divertor region are designed to continuously exhaust helium ash, along with unconsumed fuel and impurities. The gas streams from the pumps go to the Tritium Plant (integrated in the Tokamak Complex, above), where the fusion fuels are extracted for reinjection into the fuelling cycle.
Multiple technologies are involved in the extraction and separation process in the Tritium Plant, grouped into six sub-systems: tokamak exhaust processing (receives reactor exhaust and separates out impurities from the hydrogen isotopes), isotope separation (receives the purified hydrogen isotope steam and separates deuterium from tritium), storage and delivery (stores ITER fuel, whether recycled or new), atmospheric detritiation (recovers tritium from impurity gases as water), water detritiation (recovers tritium from tritiated water and returns it to the fuelling stream), and analytics (chemical and isotopic analysis in support of the five other sub-systems).