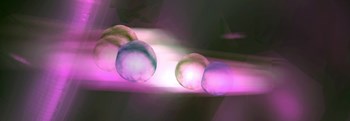
The fusion of deuterium (D) and tritium (T) atoms has been proven in the laboratory to produce the highest energy gain at the ''lowest'' temperatures.
Although different isotopes of light elements can be paired to achieve fusion, the deuterium-tritium (DT) reaction has been identified as the most efficient for fusion devices. ITER and future devices will use the hydrogen
isotopes deuterium and tritium to fuel the fusion reaction.
Deuterium can be distilled from all forms of water. It is a widely available, harmless, and virtually inexhaustible resource. In every cubic metre of seawater, for example, there are 33 grams of deuterium. Deuterium is routinely produced for scientific and industrial applications.
Tritium is a fast-decaying radioelement of hydrogen which occurs only in trace quantities in nature. It can be produced during the fusion reaction through contact with lithium, however: tritium is produced, or "bred," when neutrons escaping the plasma interact with lithium contained in the blanket wall of the tokamak.
Lithium from proven, easily extractable land-based resources would provide a stock sufficient to operate fusion power plants for more than 1,000 years. What's more, lithium can be extracted from ocean water, where reserves are practically unlimited (enough to fulfill the world's energy needs for ~ 6 million years).
Global inventory for tritium is presently around twenty kilos, which ITER will draw upon during its operational phase. The concept of "breeding" tritium within the fusion reaction is an important one for the future needs of a large-scale fusion power plant.

440 blanket modules cover the inside of the vacuum vessel and directly face the hot plasma. Certain modules in ITER will be used during later-stage operation to test tritium breeding concepts.
In the deuterium-tritium (DT) fusion reaction, high energy neutrons are released along with helium atoms. These electrically-neutral particles escape the magnetic fields that confine the plasma and are absorbed by the
blanket covering the surrounding walls.
If the blanket modules contain lithium, a reaction occurs: the incoming neutron is absorbed by the lithium atom, which recombines into an atom of tritium and an atom of helium. The tritium can then be removed from the blanket and recycled into the plasma as fuel.
Blankets containing lithium are referred to as breeding blankets. Through them, tritium can be bred indefinitely.
Once the fusion reaction is established in a tokamak, deuterium and lithium are the external fuels required to sustain it. Both of these fuels are readily available.
A future fusion plant producing large amounts of power will be required to "breed" all of its own tritium. Through its Test Blanket Module (TBM) program, ITER will be the first fusion device to test this essential concept of tritium self-sustainment.
A fusion reaction is about four million times more energetic than a chemical reaction such as the burning of coal, oil or gas. While a 1000 MW coal-fired power plant requires 2.7 million tonnes of coal per year, a fusion plant of the kind envisioned for the second half of this century will only require 250 kilos of fuel per year, half of it deuterium, half of it tritium.
Only a few grams of fuel are present in the plasma at any given moment. This makes a fusion reactor incredibly economical in its fuel consumption and also confers important safety benefits to the installation.