What will ITER do?
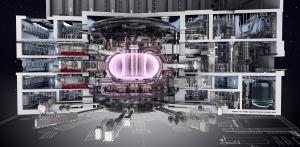
The amount of fusion energy a tokamak is capable of producing correlates directly to the number of fusion reactions taking place in its core. Scientists know that the larger the vessel, the larger the volume of the plasma ... and therefore the greater the potential for fusion energy.
With five times the plasma volume of the largest machine operating today, the ITER tokamak will be a unique experimental tool, capable of longer plasmas and better confinement. The machine has been designed specifically to:
1) Achieve a deuterium-tritium plasma in which the fusion conditions are sustained mostly by internal fusion heating
Fusion research today is at the threshold of exploring a burning plasma. In a burning plasma, the heat from the fusion reaction is confined within the plasma efficiently enough for the self-heating effect to dominate any other form of heating. As the first such burning plasma device in the world, ITER will offer scientists a unique opportunity to chart new territory in controlled nuclear fusion.
2) Generate 500 MW of fusion power in its plasma for long pulses
The world record for fusion power in a magnetic confinement fusion device is held by the European tokamak JET. In 1997, JET produced 16 MW of fusion power from a total input heating power of 24 MW (Q=0.67). ITER is designed to yield in its plasma a ten-fold return on power (Q=10), or 500 MW of fusion power from 50 MW of input heating power. ITER will not convert the heating power it produces as electricity, but—as the first of all magnetic confinement fusion experiments in history to produce net energy gain across the plasma (crossing the threshold of Q≥1) —it will prepare the way for the machines that can.
Of course, since ITER is an experimental device, the energy balance in focus in the ITER design is that of the fusion process itself. When taking into account the electricity consumption of the whole plant, the ITER design includes redundant systems, exceptional numbers of diagnostics, and other aspects that are inherently inefficient. Future commercial designs, to be successful, will need to have a favourable comparison between output fusion power and electricity production, and the total input electric power.
3) Contribute to the demonstration of the integrated operation of technologies for a fusion power plant
ITER will bridge the gap between today's smaller-scale experimental fusion devices and the demonstration fusion power plants of the future. Scientists will be able to study plasmas under conditions similar to those expected in a future power plant and test technologies such as heating, control, diagnostics, cryogenics and remote maintenance.
4) Test tritium breeding
One of the missions for the later stages of ITER operation is to demonstrate the feasibility of producing tritium from lithium (lithium isotope Li-6) within the vacuum vessel. The world's supply of tritium (used with deuterium to fuel the fusion reaction) is not sufficient to cover the needs of future power plants. ITER will provide a unique opportunity to test mockup in-vessel tritium breeding blankets in a real fusion environment.
5) Demonstrate the safety characteristics of a fusion device
In 2012, when the ITER Organization obtained licensing as a nuclear operator in France, the ITER fusion device became the first in the world to have successfully undergone the rigorous examination of its safety case. One of the primary goals of ITER operation is to demonstrate control of the plasma and fusion reactions with negligible consequences to the environment.
The phases of ITER
The ITER Members are procuring the components and systems of the ITER machine and plant and shipping them to the ITER site, where the ITER Organization teams have the overall responsibility for the successful installation and assembly of all components. (Read more about how the ITER Organization is managing machine and plant assembly here.) Systems and services will be commissioned progressively, before an integrated commissioning phase demonstrates that the all systems required for the first experimental program perform as expected. The experimental exploitation of ITER will follow.
Operation will be carried out according to a detailed Research Plan—a document that describes the progressive operation phases of ITER. In 2024, the ITER Organization and the seven ITER Domestic Agencies presented an updated project baseline (technical scope, cost and schedule) to the ITER Council for approval and, within that, a new baseline ITER Research Plan. The overall approach, which was approved, foresees a Start of Research Operation phase (SRO), will feature a machine equipped with a divertor, blanket shield blocks and other key components and systems, allowing for hydrogen and deuterium-deuterium plasmas that culminate in the operation of the machine in long pulses at full magnetic energy and plasma current. The initial phase will be followed by deuterium-tritium operation 1 (DT-1) with limited fluence, and finally a machine upgrade to enable a more extensive DT-2 phase with the target to complete all project goals including the Q=10 project specification. (See further detail in this article or this article.)