Scientists and cloud computing push the boundaries of ITER plasma disruption simulations
Scientists from the ITER Organization and the Max Planck Institute for Plasma Physics have performed the first realistic three-dimensional simulations of ITER plasma disruptions with cloud computing support in collaboration with Google. These large-scale simulations help scientists to understand the physical processes occurring during disruptions and confirm the capability of the disruption mitigation system to reduce the electromagnetic forces to the levels required on the ITER Tokamak.
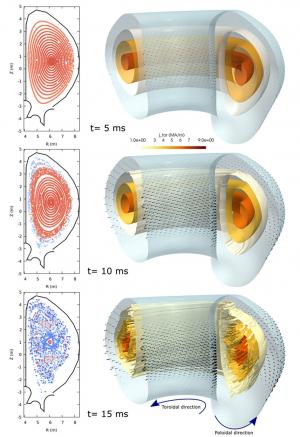
As supercomputers become more and more powerful and the fidelity of plasma simulations improves, scientists are able to make better predictions. Nevertheless, there remain particularly challenging tokamak plasma phenomena such as disruptions, in which the thermal and magnetic energy stored in the plasma is lost on very rapid timescales. Modelling these events and thus understanding better how to avoid them or mitigate their impact on tokamak operation, pushes the limits of today's simulation capabilities.
In present tokamak experiments, a disruption may occur when operating near plasma stability limits or when systems malfunction. ITER will be equipped with an extremely sophisticated disruption mitigation system to minimize the effects of these transient events on the tokamak components.
Although disruption events are rather short (typically less than 150 milliseconds in ITER), magneto-hydro-dynamic simulation codes need months of computations and millions of computer hours to simulate a few tens of milliseconds. For such tasks, supercomputers are really the only approach with which results can be obtained in a reasonable time. In a recent study, scientists from the ITER Organization and the Max Planck Institute for Plasma Physics in Germany have simulated ITER mitigated disruptions thanks to a collaboration with Google to demonstrate a cloud computing proof of concept. Extensive Google Cloud services, together with those of the ITER Scientific Data and Computing Centre and the EUROfusion Marconi supercomputer, have been leveraged to push towards more realistic plasma descriptions.
While there are still several modelling aspects to be further refined, these simulations confirm that the ITER disruption mitigation system will be able to reduce the global magnetic forces acting on the ITER vacuum vessel, blanket/first wall and divertor. Such forces arise during the loss of the plasma magnetic energy known as the "current quench" phase of the disruption, in which the plasma current decays very rapidly. During this collapse phase, electric currents induced in the vacuum vessel and in-vessel components (blanket/first wall and divertor) drive the production of magnetic forces. A description of these new results, just published in the journal Nuclear Fusion, clearly demonstrates the benefits of a fast current quench (compared to the decay time of the vessel currents) in reducing the vacuum vessel forces. The ITER disruption mitigation system will control the speed of the current quench by cooling down the plasma with cryogenic pellets. Newsline has regularly reported how this technique is being demonstrated routinely in many research tokamaks (for example in ASDEX Upgrade, DIII-D, KSTAR and JET) coordinated by the ITER Disruption Mitigation Task Force in support of the physics basis and technology design of the ITER disruption mitigation system.